Supporting Scalable Cell Therapy Using Allogeneic Workflows

Historically, cell therapies are used to treat patients with cancer after relapse from other approved treatment modalities, or if no approved treatment is available. However, the introduction of allogeneic cell therapies has created exciting opportunities to broaden access to cell-based treatments. With advancements in manufacturing, developers are becoming increasingly interested in commercializing allogenic therapies, bringing them one step closer to becoming broadly available to more patients.
Since first conceptualized in the nineteenth century, cell therapy has cemented its role in treating some of the most complex diseases and conditions. As one example, successful autologous and allogeneic bone marrow transplantation has become common practice in treating patients with leukemia, lymphoma, aplastic anemia, and immune deficiency disorders, with more than 18,000 patients receiving a bone marrow or umbilical cord blood trans-plant every year in the United States.1 Beyond this application, stem cell and cell transplantation applications have garnered increased interest in recent decades, with incredible strides made in the twenty-first century.
Among those advancements, the introduction of allogeneic cell therapies is of specific interest. In the allogeneic approach, healthy donor cells are used to develop cell therapies, rather than relying on harvesting the patient’s own cells to develop a personalized treatment. Personalized therapies—such as chimeric antigen receptor (CAR) T-cell treatment for lymphoma and leukemia—have shown incredible effectiveness, but traditional autologous workflows that rely on using a patient’s own cells can limit availability of these treatments due to the high complexity and price.
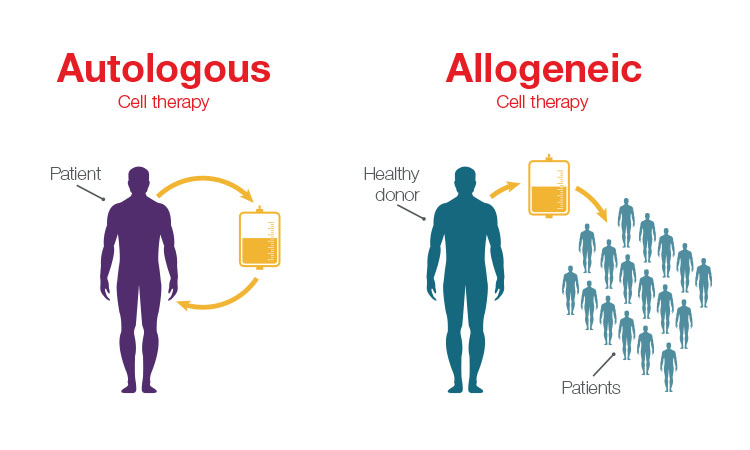
The main difference between allogeneic and autologous therapies is the source of the cells. Allogeneic therapies are manufactured in large batches from unrelated healthy donor tissues (e.g., bone marrow or from lymphocytes such as natural killer (NK) cells and T cells), whereas autologous therapies are manufactured as a single lot from the patient being treated.
Unlike autologous workflows, which offer a one-to-one patient outcome, allogeneic workflows offer increased scalability because they use cells from healthy donors to create a cell bank system that can be used to treat multiple patients. The main driver for developing allogeneic therapies is to offer considerably more cost-effective and widespread therapies to more patients.
Scalable and Accessible
Allogeneic cell therapies rely on a single source of cells to treat many patients. This automatically makes them a more scalable alternative to autologous therapies. CAR T-cell therapies offer one opportunity where more efficient development and manufacturing can make a profound impact, providing potentially more effective treatment options for some of the most vulnerable cancer patients.
The growth in CAR T-cell research is exploding. Although only a handful of cell and gene therapies are on the market, the US FDA predicts that by 2025, it will grant up to 20 cell and gene therapy approvals per year.2
Current autologous models for developing CAR T-cell therapies are time consuming: it can take three weeks on average from when the cells were first collected to treatment of the patient with a CAR T-cell therapy. This is an expensive and lengthy process with little room for error, with patients who are desperate for treatment. Researchers and organizations continue to work diligently to optimize the manufacturing process to develop safe and effective CAR T-cell therapies as efficiently as possible, in the hope that one day they can be manufactured at a lower cost and made available to any patient who could benefit from them.
Scientists are hopeful that allogeneic workflows can make CAR T-cell therapy more accessible by manufacturing therapies using healthy donor cells or induced pluripotent stem cells (iPSC)-based cell platforms.3 These can be used to treat multiple patients as an “off-the-shelf” treatment. Beyond the scale this empowers, these healthy T-cells are in better condition than the patient’s own cells to be reprogrammed, proliferate, and injected into the patient to fight the cancer.
Materials and Manufacturing Innovations
Allogeneic therapies can be more cost effective because they can be mass produced to treat multiple patients, but the workflow is still time consuming. Just as with autologous models, it takes three weeks or more from cell collection to development and administration of allogeneic cell therapy. But, as technology companies, in collaboration with drug developers, continue to design fit-for-purpose workflows and solutions designed specifically for the development of allogeneic cell therapies, this approach will continue to gain steam and show comparable or even favorable improvements over autologous models.
As one example, Thermo Fisher Scientific has developed a new xeno- and serum-free cell culture media specifically suited for allogeneic T-cell manufacturing using healthy donor materials. The media’s formulation is designed to increase the drug product yield by extending the duration of the manufacturing time (Thermo Fisher Scientific unpublished data), while maintaining the desired T-cell phenotypes that are demonstrated to correlate with favorable clinical responses.4
The importance of cell culture media has been reported by researchers in the field. According to a recent article in the Journal of Clinical Hematology, “Subtle changes in media formulation such as changing protein source from human serum to serum-free, concentrated, platelet extract has a dramatic impact on the effectiveness of T-cell therapies.”5
Similarly, innovations in manufacturing are enabling a fundamental shift in the scale of cell therapy production, ultimately helping a greater number of patients benefit from these therapies. Careful selection of healthy donor T-cell materials for manufacturing allogeneic therapies may contribute to a more robust manufacturing of the drug by minimizing the challenges related to the considerable biological variability seen in autologous T-cell materials, which often have a history of previous chemotherapies and stem cell transplantation. Setting up a reliable, consistent, and scalable manufacturing system is key to producing cell therapies on a wider scale.
Closed-system solutions also promise to improve the efficiency and safety of cell processing operations. 6 Cell therapy is a complex and labor-intensive process that can require open manipulations that can introduce process variability and/or contaminants. Processing involves multiple steps and procedures including cell isolation and activation, gene editing, cell expansion, and wash and concentration. For a successful, commercially feasible cell therapy process, it is important to be able to conduct these activities as simply, safely, and efficiently as possible. Closed systems provide the highest containment and product safety protection for these fragile substances.
Using equipment and products that scale from research to manufacturing can cut process development times and delays by eliminating the need to learn and optimize new systems while also permitting multiple batches to be processed in a shared clean room. Removing human involvement and error from this process further supports consistency of both autologous and allogeneic therapies by creating a reproducible and automated manufacturing workflow for these therapies.
Risks and Challenges Ahead
Thanks to recent advancements, cell therapies are receiving increased attention: the Alliance for Regenerative Medicine’s Annual Report 2020 lists more than 1,200 active, or actively recruiting, clinical trials for cell therapies being developed for diverse diseases.7 But only a handful of cell therapies have been approved and are on the market. Although this field is continuing to expand, thanks to the innovations highlighted previously, challenges remain that must be addressed.
When working with allogeneic therapies, there is an increased risk in some applications (e.g., T-cells) of immunological response from the patient that could be life-threatening, such as severe graft vs. host disease. Because these situations use donor cells instead of the patient’s own cells, new technologies are emerging to address and prevent these life-threatening events. Clustered regularly interspaced short palindromic repeats (CRISPR) and transcription activator-like effector nucleases (TALEN) gene-editing technologies are used to address the safety concerns related to using donor T-cells. Additionally, a purification step is often taken to remove the remaining donor T-cells to ensure a safe drug is delivered to patients.8 Purification steps are routinely used in cell therapy manufacturing, using magnetic beads conjugated with specific antibodies that isolate specific immune cells for further drug manufacturing or to remove unwanted cells from the drug product. CRISPR-based technologies are also under testing in clinical trials to advance the treatment of inherited genetic diseases (e.g., beta thalassemia) and CAR T-cell therapy.
On the other hand, NK cells, which are a type of lymphocyte (a white blood cell) and a component of innate immune system that play a major role in the host-rejection of both tumors and virally infected cells, are emerging as a “natural” allogeneic alternative to the use of donor T-cells. There are few-er safety concerns to manage with NK cells. Recently, chimeric antigen receptor (CAR)-NK cells have shown promising clinical responses in the treatment of CD19-positive blood cancers.9 Due to the increased risk and safety profile in certain allogeneic platforms, it is expected that regulators are looking for extensive safety documentation before we will see such therapies approved.10
Regulatory Hurdles
Another key challenge associated with cell therapy of any kind—not just allogeneic therapies—is related to regulatory guidance. Cell and gene therapy in the US is under the FDA’s Center for Biologics Evaluation and Research (CBER). Regulations for cell and gene therapies were established relatively recently and are still evolving. There are efforts underway to harmonize the global regulations of cell therapies.
Because cell therapy can be influenced by differences in donor and tissue sources, product characteristics may not be well defined in early clinical stages of development, making them harder to regulate. Identification of relevant biomarkers remains a challenge that could allow for the selection of donor materials with an expected cell therapy drug response and biomarkers to monitor the quality attributes during the manufacturing of the drug. Among other challenges are the issues with analytical testing, such as assay specificity, sensitivity, accuracy, and reproducibility, which can be important for demonstrating batch-to-batch consistency and product comparability. Early and frequent collaboration with regulatory agencies during both the approval and development phases is paramount.
In allogeneic cell therapies, specifically, there are robust requirements to maintain traceability from donor to recipient once the therapy has been delivered. There are additional guidelines that need to be followed to minimize the risk of transmission of diseases (e.g., through viruses) from the donor to the recipient.11 For example, determinants of donor eligibility should ensure that the unrelated allogeneic cells or tissues are obtained from donors who have been appropriately screened and tested for identified communicable diseases that may arise from viruses and bacteria.
These regulations are continuing to move forward in both the development and delivery of these therapies. Ultimately, as technology evolves to make these processes more safe, scalable, and reproduceable, we can expect to see openness from regulators to embrace these systems.
Conclusion
There is huge promise around allogeneic therapies, and scientists and regulators are well on their way to addressing some of the major challenges and making these therapies a more widely available option for patients. Innovations to optimize and standardize the workflow will go a long way toward supporting scalable cell therapy development and manufacturing. Just as cell therapies have allowed us to activate cells for a medical effect, allogeneic workflows can deliver these results for a larger percentage of patients. In the future, we can expect to see expanded applications for cell therapies.