Oligonucleotides: A Cornerstone for Therapeutics & More

Resounding clinical successes and maturation of extensive therapeutic pipelines have catapulted oligonucleotides from a fringe modality to therapeutic relevance in just a few short years. Oligonucleotides are a cornerstone of a burgeoning class of drugs classified as nucleic acid therapeutics. These therapies interact with DNA and RNA targets rather than traditional protein therapeutic targets. Oligo therapies offer access to gene regulation mechanisms that were previously inaccessible for treatment. Oligos are also a key component of gene editing systems, serving as the guiding instructions for DNA and RNA editing technologies.
The oligonucleotide therapeutics industry has been around since the mid-1980s but had a period of relative stagnation for years. Much of the 2000s and 2010s were fraught with clinical setbacks and companies exiting the space. A few innovators have shepherded this industry through these down years and managed to build impressive pipelines. Since 2016, drug approvals per clinical campaign have outpaced the average across all modalities, resulting in 11 FDA-approved therapies in that span. This platform technology is being adopted by growing ranks of large pharmaceutical manufacturers via licensure and acquisitions and by dozens of innovators. As of earlier this year, 80 molecules were in phase II and phase III clinical trials, with many more molecules in development.1 There has been significant global interest in investment to rapidly expand commercial and clinical manufacturing capacity.2 The industry is responding to the lack of manufacturing capacity with creative science and engineering solutions.
Overview
The oligo manufacturing platform has been developed based on solid phase synthesis, which consists of a series of chemical reactions that covalently link modified nucleotides to create a molecule of appropriate length, all while anchored to a solid support. Synthesis is typically followed by a cleavage reaction, which cleaves the molecule of interest from the solid support, and a subsequent deprotection reaction, which removes protecting groups and renders the molecule biologically active. Typically, the drug substance is then purified via one or more chromatography steps, concentrated via ultrafiltration, and isolated via lyophilization. The active pharmaceutical ingredient (API) drug substance (DS) from lyophilization is then transported to a fill/finish facility for final formulation and filling into vials for sterile injection. Figure 1 shows a typical block flow sequence of unit operations. In this article, we’ll explore some innovations and improvement opportunities in the science, manufacturing processes, and facilities that produce these therapies.
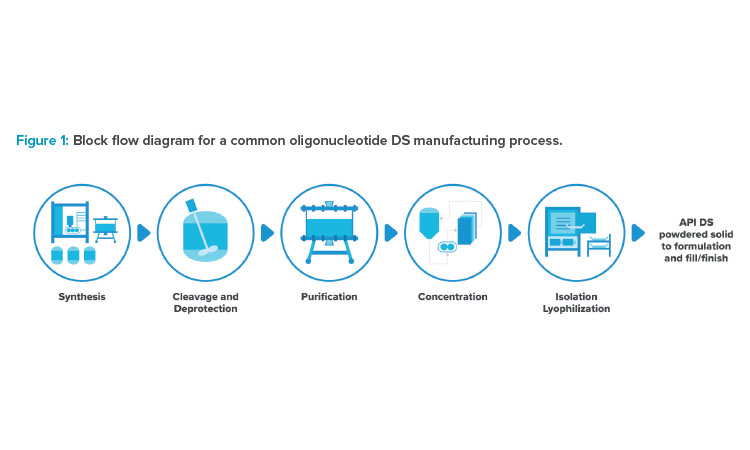
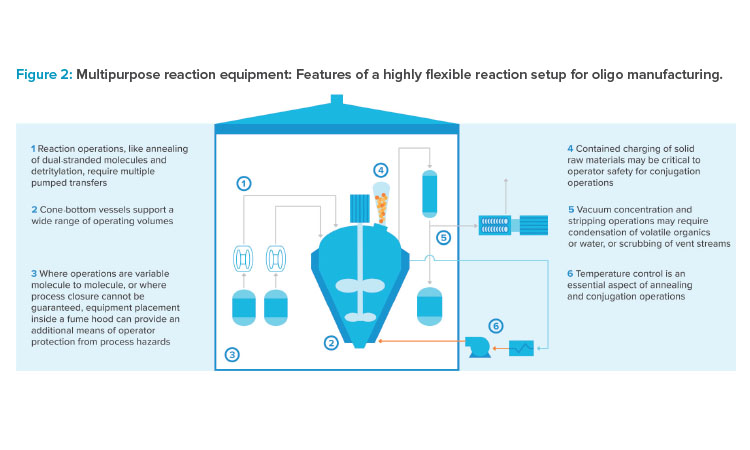
Synthesis and Reaction
The solvent burden in oligonucleotide production is significant, owing in large part to the solid phase reaction platform where reactants are repeatedly flushed from the synthesis column. Solvent usage per kg of API produced can easily exceed 3,000 liters, much of which is acetonitrile. The demand for acetonitrile from the oligonucleotide industry may soon outpace the global production capacity, so reduction of solvent usage is a point of emphasis.
One approach to reducing solvent usage is to reduce the dose size of therapies. There have been promising developments in the industry to maximize the effect of these molecules without parallel increases in toxicity. Nucleotide modifications, whether by alteration of synthesis starting materials or alternate reactive chemistries, can help achieve this goal. Experimentation with mesyl backbone modifications has demonstrated a significant broadening of the therapeutic index in preclinical studies.3 Wave Life Sciences has likewise reported advantages with a nitrogenated backbone, and Alnylam has demonstrated promising results with (E)-vinylphosphonate backbones.4, 5 Many innovations in base chemistry have been developed and implemented in recent years; a notable example is bridged/locked nucleic acids (BNA/LNA), which confer increased stability to molecules. The full clinical benefits of these chemistry advancements have yet to be realized, but there is tremendous promise.
For many of these cases, the production nuance all exists in the starting materials, where convoluted manufacturing processes drive up pricing but produce starting materials using classical reaction and isolation equipment via organic chemistry. These high-value molecules can then be integrated into a synthesis cycle with ease via a simple substitution of reactant solutions. However, the use of new base chemistries sometimes requires unique engineered solutions. In the case of mesyl azide, liberated nitrogen during the coupling reaction must be removed from a closed, pressurized system and excess reactant requires quenching prior to disposal, resulting in a need for specialized equipment upstream and downstream of the synthesis column.
Other work seeks to overcome drug delivery challenges to limit dose sizes. Significant investment has been made in expanding the targeted delivery of oligos to muscle, heart, lung, skin, and brain tissues, among others. Conjugation of oligos to targeting ligands such as peptides, antibody fragments, aptamers, and proteins can unlock these areas of disease relevance to access disease targets that were previously difficult or impossible to reach. Endo-somal escape mechanisms are also being utilized to enhance bioavailability of these medicines. Studies have shown that a vast majority of an administered siRNA or antisense oligonucleotides (ASO) dose that is transported across the cell membrane is trapped and degraded prior to exiting the endo-some, thus never reaching its target.6
Engineered molecules with a knack for breaching endosomes are being developed to significantly increase the proportion of a dose that has therapeutic effect. These innovations require a diverse chemical manufacturing toolbox, elements of which are displayed in Figure 2. Stirred tank reactors are needed to forge covalent linkages between oligonucleotide and targeting ligands. Broad pipelines require facilities to be designed with a large degree of flexibility to support anything from mild reaction conditions to the use of potent or highly biologically active compounds. Large walk-in fume hoods are organized to support a variety of unit operations. Cone-bottom reaction vessels can provide the turndown required to process a variety of scales and handle different reactions.
Purification and Isolation
Purification of oligonucleotides generally results in multiple eluate fractions of inconsistent purity and yield. As a result, analytical timelines to verify eluted purity and concentration of full-length product often bottleneck facility production. To combat this, facilities must be designed with appropriate capacity to handle the intensive analytical burden, and additional tankage is often required for simultaneous in-process storage of multiple eluate batches.
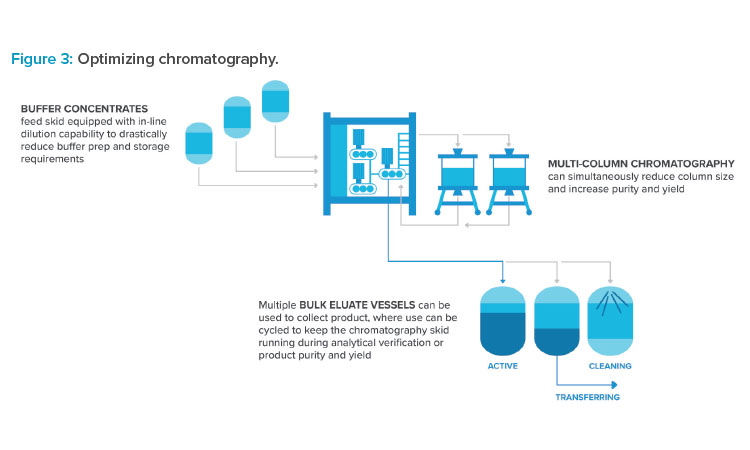
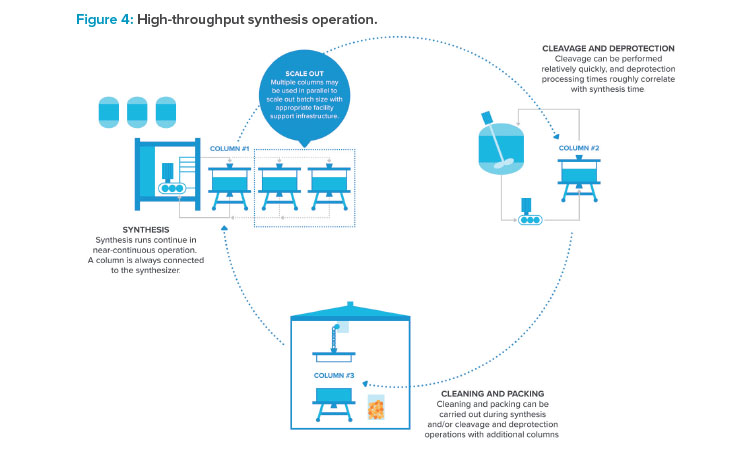
Many innovations commonly adopted in biologics production are starting to find footing in oligo facilities. Use of buffer concentrates combined with inline dilution allows a significant reduction in capital equipment to support high-throughput chromatography and ultrafiltration operations. Buffer tank sizes can be reduced significantly or eliminated entirely. Multicolumn chromatography systems are receiving increased attention to drive to higher puri-ties and yields. Some systems are designed to recycle off-peak side fractions that are normally discarded, increasing yield.7 Ultimately, focused process development is required to take advantage of these opportunities. Figure 3 highlights opportunities for optimizing chromatography.
Lyophilization
Lyophilization has been the classic unit operation of choice for isolation of oligonucleotides prior to formulation and filling. Unfortunately, lyophilization is energy intensive and time consuming, often requiring 3 to 5 days to complete a batch. Oligos generally have excellent stability in aqueous solutions, and lyophilized oligos are dissolved in aqueous formulation buffers for preparation of the final dosage form anyway. As a result, some companies have challenged the paradigm by forgoing lyophilization entirely, opting to provide solution phase API to drug product facilities for filling and packaging. Some regulatory hurdles remain around classification of solution phase oligo as API versus drug product intermediates.8
Getting More from Facilities
Lack of manufacturing capacity on the market encourages the intelligent use of a facility that removes bottlenecks from production schedules and ensures high utilization of high capital cost equipment. Maximizing production of a synthesizer is essential. Figure 4 highlights opportunities to do just that. Synthesis with multiple columns in various stages of processing (packing, synthesis, and cleaning) can maximize equipment occupancy. Synthesis columns have historically been limited in size due to weight and bulk as well as effectiveness of column seals. Synthesis using multiple columns in parallel can allow for larger batch sizes. Rotating multiple columns through various stages of packing, synthesis, cleavage and deprotection, and cleaning can allow for synthesis to be essentially continuous.
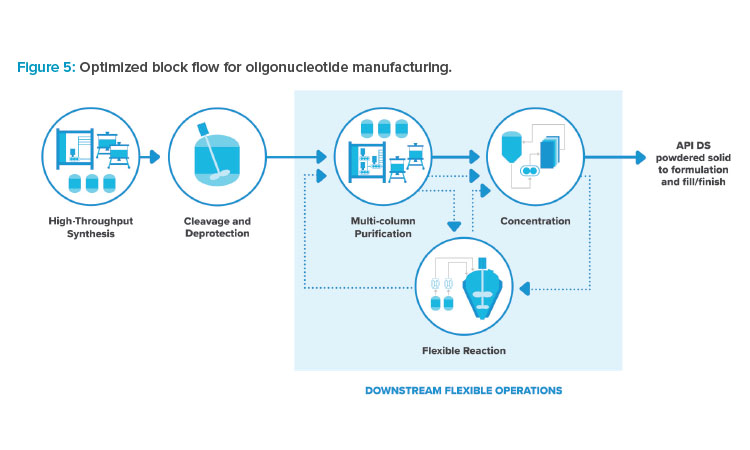
The synthesis unit operation routinely takes 8 to 12 hours start to finish, but it can take 3 to 5 days between batch starts using a single synthesis column. Cycling through columns can drastically increase synthesis output.
Combining the incremental process improvements previously mentioned results in an optimized facility, with the adjusted block flow shown in Figure 5. Multiple alternate flowpaths exist between downstream operations, as indicated by the dotted lines. The facility footprint is focused on high throughput and high utilization of expensive process skids. The facility provides a high degree of processing flexibility to account for the variation in molecules and delivery technology, producing liquid phase DS at high purity and yield.
Redefining the Standard
Companies have been looking for scalable alternatives to solid phase synthesis in hopes of drastically reducing solvent usage, whether through adaptation of existing biological mechanisms for nucleic acid production or completely novel innovation. Enzymatic synthesis techniques are being explored in the hope that this field’s future may look like that of mRNA synthesis, where relatively small batch reactors can produce large quantities of mRNA via assembly of unmodified nucleotides with polymerases and template RNA.
Significant barriers remain to applying this biological approach to oligonucleotides, which are, by their nature, heavily chemically modified for nuclease resistance. At scales approaching tens of kilograms per batch, liquid phase convergent chemical synthesis may prove to be an attractive option. This approach would link fragments 3 to 5 nucleotides long, where the fragments could be manufactured at large scales. This could eliminate the need for highly specialized equipment during synthesis, instead using batch reactors.9 Ligation of duplex molecules such as siRNA from several starting mate-rial fragments is being explored as another approach to convergent synthesis. Any successful enzymatic approaches could augment the efficiency of convergent synthesis.
This article primarily addresses manufacture of therapeutic targets at a length of 18-30 nucleotides per molecule, which encompasses most siRNA and ASOs. These lengths confer good overall reaction yields while utilizing solid support that can be densely loaded. Synthetic guide RNA (sgRNA) molecules are a notable exception to this category of oligos. With lengths often approaching or exceeding 100 nucleotides, sgRNA manufacture by solid phase synthesis exacerbates the problem of high solvent usage; longer synthesis cycles require an increase in reagent and solvent usage proportional to the increase in length.
That solvent usage per gram of usable product is further impacted by reduced overall yields of full sequence length molecules due to accumulated incremental yield loses per coupling. Solid supports for sgRNA have much lower loading relative to shorter molecules due to steric interference at link-age sites, resulting in less moles of product per unit volume of synthesis column. This in turn increases solvent usage per cycle relative to a shorter molecule due to rinse efficiency of the proportionately larger volume beds per gram produced. This increase results in sgRNA manufacturing facilities having a disproportionate solvent and reagent support infrastructure relative to that of an ASO or siRNA manufacturing facility at similar batch sizes.
Conclusion
The recent expansion of global oligo manufacturing demand has presented numerous opportunities to design better molecules, better processes, and better facilities. Better molecules are being designed by taking advantage of chemistry to reduce toxicity, increase bioavailability, and promote interaction with unique cell types. Better processes are being designed to produce more material faster, at lower cost, driving efficiency for these high-value facilities. Better facilities are being designed to increase flexibility to accommodate these process and chemical improvements, to handle variation in chemistries, and to increase throughput. The future for oligonucleotide therapies, and the patients who will receive this combination of best-in-class and first-in-class treatments, is undeniably bright.