Life-Cycle Approach to Cleaning Topical Drug Products

Topical drug products and cosmetics are often manufactured in the same facility under a unified quality standard that supports the topical drug products’ performance and label claims. Cleaning is an important component of a manufacturing process, and the process life-cycle approach should be followed for cleaning validation.1, 2 This article explores the life-cycle approach to cleaning topical drugs and cosmetics with attention to the cleaning design phase and leveraging this information, including lab studies and pilot runs, for qualifying and monitoring the cleaning process.
The ideal case is a site using one cleaning procedure with an acceptable set of critical cleaning parameters (CCPs) for all products. However, some of the products manufactured in a topical drug facility can be difficult to clean. For example, the equipment for blending and packaging large-volume, high-viscosity formulations is complex, and the residues can be challenging to remove. Therefore, understanding and designing an effective cleaning process through laboratory testing, field trials, equipment designed for cleanability review, and risk assessments is critical to reducing resources and costs associated with cleaning validation and monitoring activities at the facility.
The cleaning validation life-cycle approach consists of three stages: design, qualification, and continued verification.3, 4 Stage one: design, includes cleaning agents and suppliers, critical parameters and cleaning methods, laboratory and pilot testing, utility considerations, process equipment design review, cleaning process map, analytical test method validation, residue limits, visual inspection, and operations partnership.
Often referred to as the validation stage, stage two: qualification confirms that the cleaning procedure under normal conditions meets preestablished acceptance criteria.5 It includes cleaning validation master plan, product risk assessment, utility and equipment readiness, analytical method readiness, sampling site selection/grouping, standard operating procedures, validation protocols, execution of validation protocols, personnel training, and the validation documentation package. Stage three: continued verification includes periodic review, process control, continuous monitoring, preventive maintenance, and periodic revalidation (if applicable).
The cleaning process design involves reviewing the equipment, utilities, wastewater concerns, nature of residue, selection of cleaning agent, cleaning parameter, analytical method, and sampling method. Application of laboratory testing and field testing can be used to determine why selected conditions are used for the qualification stage but not the monitoring stage. Various CCPs may include, but are not limited to, cleaning concentration, temperature, wash time, water quality, surface material, and dirty hold time.
This article explores the life-cycle approach to cleaning topical drugs and cosmetics with attention to the cleaning design phase and leveraging this information, including lab studies and pilot runs, for qualifying and monitoring the cleaning process.
Cleaning Process Design
Cleaning Agents and Suppliers
Topical drugs and cosmetic products contain a wide range of components based on the desired properties of the products. These components can have poor solubility in solvents such as water and can be challenging to clean. Choosing an appropriate cleaning agent and supplier is critical to having an effective and efficient cleaning process. Like cosmetics, cleaning agents may be formulated chemistries that may contain several components to ensure broad effectiveness. They may, for example, contain components such as surfactants and chelants to help remove insoluble residues and drug actives to acceptable limits. It is also essential to understand the cleaning agent rinse profile, analytical methods for residue detection, and detergent toxicity profile. The supplier’s manufacturing process should provide a consistent product, quality expectations, and technical assistance to support the cleaning agent’s application within cGMP facilities.
Critical Parameters and Cleaning Methods
Laboratory testing is the first step in defining the critical parameters for removing process residues before materials are taken to the manufacturing floor. Critical parameters include time, action, concentration, and temperature (TACT), as well as water quality, surface, soil load and condition, and environmental factors.6 When designing the study, it is crucial to mimic plant conditions and restrictions as accurately as possible and to consider the method of cleaning. Most cleaning under consideration is for a clean-in-place (CIP) application, which consists of a spray impingement and cascading flow in the production vessel and turbulent flow through pipes and cleaning circuits.7
The following procedure is used throughout the studies:8
- Step one: Dry, clean 304 stainless steel coupons (7.5 × 15 cm size) with a 2B finish are weighed on an analytical balance (±0.1 mg) to obtain the pre-coating weight. This step establishes a baseline weight for the coupon, and will help determine how much residue should be coated on the coupon. In addition, the weight of the dried coupon will be used for comparison to all future weights after a simulated cleaning process.
- Step two: The coupons are coated with samples. The amount of residue per surface area is controlled and recorded.
- Step three: The conditioned coupon is weighed on an analytical balance to determine the pre-cleaning weight.
- Step four: Each coupon is cleaned with agitated immersion, spray wash, and cascading flow. Agitated immersion testing is usually the best method to determine cleaning chemistry, concentration, time, and temperature. The primary cleaning effect is caused by the chemical action of the cleaning agent. Spray wash is performed in a modified washer/disinfector or washer at 76 kPa (approximately 11 psi). Cascading flow is performed at 2 L/min (approximately 0.5 gal/min). These procedures simulate the expected cleaning process. If needed, higher pressure or flow rate is evaluated. If a spray ball apparatus is used for cleaning, spray impingement and cascading flow represent spray ball cleaning.
- Step five: Each coupon is removed at selected time points and visually observed for cleanliness.
- Step six: Each coupon side is rinsed with tap water for 10 seconds at a flow rate of 2 L/min. The temperature of the tap water is 20°C to 25°C unless specified otherwise.
- Step seven: Each side of the coupon is rinsed with deionized water and examined for a water-break-free surface. Water-break-free is a qualitative test that indicates the cleanliness of a metal surface. On a clean surface, free from organic residue, water sheets evenly—without any breaks in the water film as it runs from the surface of the metal panel.
- Step eight: Coupons are dried and then weighed on an analytical balance to determine the post-cleaning weight. The coupon is clean if it was (a) visually clean and (b) water-break-free, and (c) if its pre-coating and post-cleaning weights are equal (0.0 mg residue).
Laboratory and Pilot Testing
With multiple, complex products manufactured on the same equipment, the approach is to choose the most difficult to clean products to help narrow laboratory testing to approximately 25–30 samples. The primary benefit of lab testing is to provide a starting point for CCPs (action, temperature, time, and concentration). These data become the basis for the cleaning cycle development at the pilot scale. An additional benefit of lab testing is to enable the initial creation of a grouping strategy or product families for pilot-scale work. Because there are products with active pharmaceutical ingredients (APIs) in the families (FDA-regulated topical drug products), the grouping strategy helps in the later stages of this work’s validation phase.
A 125 L pilot unit of the Symex system is used for development work to enable scaleup to the production systems. Given the short development window of six months, a parent-child model is used for our product portfolio of more than 300 products. The 300 products have been narrowed down to about 60 selected products during the developmental phase and grouped into families. The goal in the design phase is to create a program that would allow for a seamless transition to validation and monitoring.
Utility Considerations
The system’s key utilities are city water (hot and ambient), purified water (ambient), steam (heating the system), chilled water (cooling the system), and compressed air (controlling valves). The cleaning cycle uses city water (process water) for the initial rinse, wash, and sanitization cycles. The post-sanitization cycle uses purified water to heat the system for the final vacuum drying step.
The system is piped for hot water and city water because some products respond better to a cold-water rinse. The city water and purified water are controlled to within 1% of the set point. The other key utilities—steam (40 bar) and chilled water—are controlled within a range of 5% around these values. Heating and cooling of the system are kept to within +/- 3°C of the set point. A clean compressed air pipe to the system controls the operation of multiple valves. The dosing of water is controlled within a 1% v/v cleaning solution range. Steam pressure is at 40 bar, and the chilled water temp is controlled within a 5% range of this.
Sample | Cleaner | Cleaning Method |
Concentration | Time per Cleaning Method (min) |
Visual Observations |
Water- Break- Free |
Temp (°C) |
---|---|---|---|---|---|---|---|
Mineral Sunscreen |
Formulated cleaner containing potassium hydroxide and detergent additive |
AI, SW, CF | 3% v/v + 3% v/v | 45 | Visually clean | Yes | 80 |
PGL Polymer | Formulated cleaner containing potassium hydroxide and detergent additive |
AI, SW, CF | 5% v/v + 5% v/v | 60/30/60 | Visually clean | Yes | 80 |
Allianz OPT | Formulated cleaner containing potassium hydroxide and detergent additive |
AI, SW, CF | 3% v/v + 3% v/v | 60/30/45 | Visually clean | Yes | 80 |
Deodorizing Body Spritzer |
Formulated cleaner containing potassium hydroxide |
AI, SW, CF | 2% v/v | 15/3/30 | Visually clean | Yes | 60 |
Perfume Compound |
Formulated cleaner containing potassium hydroxide |
AI, SW, CF | 1% v/v | 10 | Visually clean | Yes | 60 |
Waterproof Mascara |
Formulated cleaner containing potassium hydroxide and detergent additive |
AI, SW, CF | 2% v/v + 2 % v/v | 60/45/60 | Visually clean | Yes | 60 |
Foundation | Formulated cleaner containing potassium hydroxide and detergent additive |
AI, SW, CF | 2% v/v + 2% v/v | 60/30/60 | Visually clean | Yes | 60 |
Serum | Formulated cleaner containing potassium hydroxide and detergent additive |
AI, SW, CF | 2% v/v + 2% v/v | 15/15/15 | Visually clean | Yes | 60 |
Concealer | Formulated cleaner containing potassium hydroxide |
SW (52psi) | 3% v/v | 45 | Visually clean | Yes | 80 |
Lip Gloss | Formulated cleaner containing potassium hydroxide and detergent additive |
SW, CF | 2% v/v + 2% v/v | 75/60 | Visually clean | Yes | 80 |
Deep Cream | Formulated cleaner containing potassium hydroxide |
SW (52 psi) | 3% v/v | 15 | Visually clean | Yes | 80 |
Foundation | Formulated cleaner containing potassium hydroxide |
SW (52 psi) | 3% v/v | 30 | Visually clean | Yes | 80 |
Gel Cleanser | Formulated cleaner containing potassium hydroxide |
AI, SW, CF | 1% v/v | 15/15/15 | Visually clean | Yes | 60 |
Clear Proof Face Mask |
Formulated cleaner containing potassium hydroxide |
AI, SW, CF | 5% v/v | 90/30/60 | Visually clean | Yes | 80 |
Process Equipment Design Review
The pilot system has numerous capabilities within the primary operations of mixing, heating, cooling, induction, pressure, vacuum, and CIP. The main vessel for primary mixing and an auxiliary vessel for additional product phases or premixes are made of 316 SS with a specified roughness (RA) finish. The transfer from the auxiliary vessel to the main vessel can be done using a pump or a pressure differential between the tanks. The system has fixed piping except for the raw material induction, which uses flex hoses. The system was designed with sanitary fittings and gasket materials that are compatible with the cleaning chemistry.
The mixing elements consist of the central agitator, outer scrapers, and homogenizer, all of which provide the system’s flow and shear characteristics. There is an additional short and long loop in this system to enable batch turnover. The short loop goes from the homogenizer to the vessel’s bottom and the long loop enters about two-thirds of the way up the vessel. The induction ports for both the main vessel and phase vessel are located on the front panels.
From a capability standpoint, the phase vessel can induct liquids, powders, and pellets. The main vessel can induct via the liquid port (into the homogenizer well), the powder port (into the homogenizer well), and the bottom port (into the vessel bottom dome). This system can work in both manual mode (semi-auto) or automatic mode (auto). A batch recipe system allows creating programs for both batch making and CIP. The human–machine interface (HMI) is located on the floor level and the platform for ease of access and operation.
The CIP chemicals are added manually after the water is dosed. The homogenizer works as the pump for the system, circulating fluid to the spray balls. The phase vessel is fitted with two cascade-style spray balls, and the main vessel is fitted with four cascade-style spray balls and side spray jets. For the main vessel, the system controls the pressure to the spray balls via a set point with a maximum value of 2,500 mbar. The vessels were tested for spray coverage during the site acceptance test (SAT) using a riboflavin test. The flow through the secondary loops in the system, like the induction and transfer lines, is also controlled by the homogenizer speed and can be customized to ensure turbulent flow. During the development run, a borescope was used to inspect the lines to determine visual cleanliness. The internal walls and mixer blades were inspected visually using a flashlight.
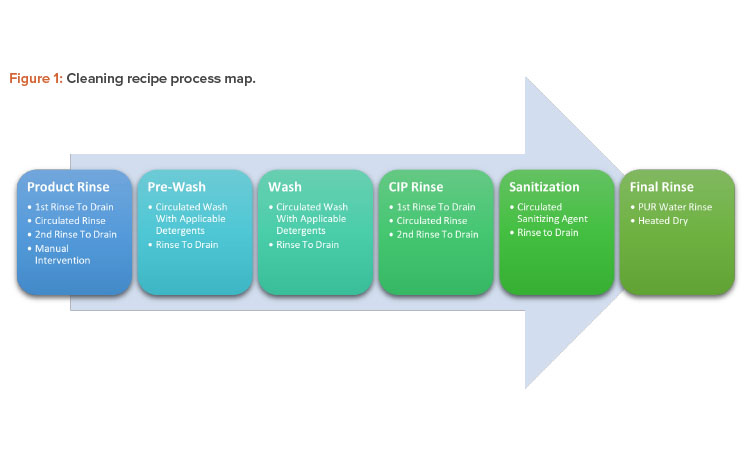
Product and Equipment Grouping
Product and equipment grouping is carefully reviewed; a well-justified grouping strategy can reduce time, personnel resources, and costs. Most of the mixing tanks are similar in design and function and are grouped. The product grouping or family is primarily based on the product chemistry. Risk ranking has the following factors included: solubility, toxicity, and hardest to clean. Product families A–H are listed next. This list is constantly reviewed when new products are commissioned into the production facility. The hardest-to-clean product from the risk ranking is defined. The residue characteristics after the dirty hold time and during the cleaning process are also considered in the risk ranking process. Different steps of the cleaning process are : Family A: surfactant systems; Family B: silicone–water systems; Family C: oil–water emulsions that needed heating; Family D: high wax anhydrous systems; Family E: high TiO2 systems; Family F: oil–water emulsions with no heating; Family G: high clay and oxide systems; and Family H: carbomer systems.
Cleaning Process Map
The cleaning recipe will follow a preset series of steps whose critical process parameters vary according to the difficulties of the related product. Figure 1 shows the process map for these steps; Figures 2 and 3 show before and after cleaning.
Analytical Test Method Validation
It is important to note that if the product and detergent residue limits provided by the calculations are high, swabbing limits will default to the upper limit of the validated test methods. For this reason, it is vital to develop testing methods that suit the processes and are flexible enough to cover a broad, extensive range that includes the residue limits that result. Another way of assessing the need for test methods is whether the resulting limitations warrant swab testing, in which case, other overarching methods can be favored. A more holistic approach to testing for residues is total organic carbon (TOC) testing if purified water is available. TOC testing can be much more reliable and sensitive for products containing organic carbon forms and is widely used in the biopharmaceutical industry.
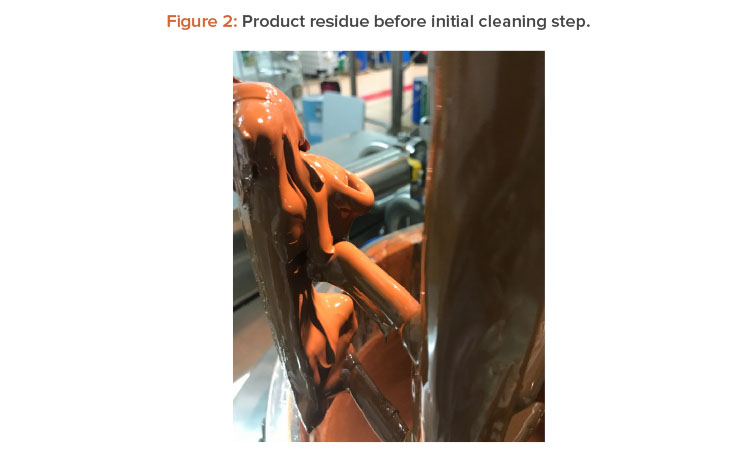
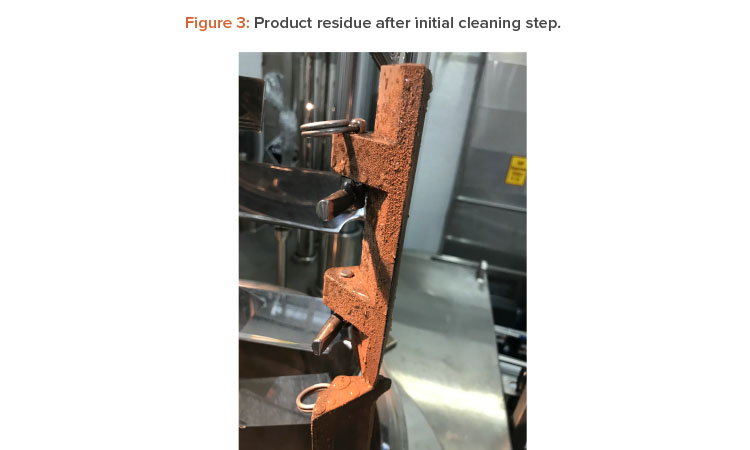
A risk-based matrix approach can be used to determine the worst-case product for each cleaning procedure.
Residue Limits
Topical drug and cosmetic products represent some of the hardest-to-clean formulations. Additionally, it is important to keep in mind the level of toxicity of these formulations. Toxicity levels and acceptable degrees of residue buildup within the system are two of the most critical drivers for assessing acceptable limits. The low toxicity of most over-the-counter active ingredients makes their impact in the development process negligible, as represented by sunscreen actives and salicylic acid. As is the case of a product whose API is not significant, the carryover concern larger risk is attributed to product buildup.
Visual Inspection
Of all the active ingredients, raw materials, and detergents used within the manufacturing facility, the alkaline cleaning detergent has the highest reported toxicity, with a permitted daily exposure (PDE) value of 7 mg/day. A PDE of this level of toxicity in consumer products results in acceptable residue limit calculations in the tens of thousands, making the visual inspection a key element in the validation process. Current literature suggests that most solid residues can be visually detected at levels of 4 µg/cm² or less, making this method the most cost-effective tool in the topical drug and cosmetic cleaning process arsenal.9, 10
Operations Partnership
Process control, training, and awareness are key elements to any successful cleaning program. Implementing a new program within a site that is manufacturing topical drugs and cosmetics requires continuous training and personnel assessment to ensure that all controls are successfully maintained. Controls tying product formulations to cleaning recipes are vital because equipment damage—rather than product contamination—is a greater concern with cleaning low-toxicity formulations.
Cleaning Process Validation
Cleaning Validation Master Plan
A cleaning validation master plan should be developed during the process development phase to guide the validation process, much like it is used for product process validation. This plan should contain, at a minimum, guidance on the following topics:
- Equipment description and system boundaries
- Product family descriptions and related cleaning processes
- Risk assessment and rationale for family groupings
- Resulting validation strategy, sampling site designation, and execution requirements
- Test method validation references
Residue limit calculations can be included in this document if the strategy focuses on a holistic set of calculations.
Product Risk Assessment
A risk-based matrix approach can be used to determine the worst-case product for each cleaning procedure. This approach considers the categories of risk factors such as ease of cleaning, solubility, toxicity, and concentration of active ingredients. Each product is rated per category according to these criteria, and each criterion has a range that defines the associated level of risk (none, low, medium, or high). Each risk factor category is assigned a multi-plier that provides weighting based on the factor’s criticality to the outcome of the cleaning process.
This assessment should be done per each product family and should be specific to the system geometry in which the cleaning process is to be executed because the cleanability of a product can vary substantially based on equipment capabilities.
System Readiness
Within a new facility, it is optimal to complete cleaning process validation alongside production process validation. This will ensure that all equipment is qualified and ready for use, as well as that no regulated products are released without first having an associated and assessed cleaning process in place. At this point, standard operating procedures should be established because of the initial development, production, and cleaning processes. Triaging parts and chemicals, manual processes, and CIP processes should have a base standard that can be reviewed as needed during and after the validation process.
Before going into a process validation phase, it is strongly advised to have first observed several runs of worst-case products. The validation stage includes successfully executing validation protocols that meet predetermined acceptance criteria. Returning to a development process within the execution of a validation protocol can come with complexities in documentation and regulatory compliance.
Continued Process Verification
Each cleaning procedure should be verified at least once every three years, or as determined by site policies, to ensure consistency and repeatability and to identify any issues that may be trending the process out of control before product contamination or finished good failures happen. The trending of critical parameters—such as cleaning agent concentration, cleaning time and temperature, and rinse water conductivity—is an important element of continued verification of the cleaning process. Each verification should be conducted in the same fashion as the previous validation process. An exception to this is when process updates are desired. A draft of these updates should be provided with the validation documentation and implemented upon successful execution.
Conclusion
The nature and quantity of topical drug and cosmetic products manufactured onsite and the desire to have a compliant, lean, and efficient cleaning strategy warrant a structured approach to cleaning. Implementing a cleaning life-cycle approach with attention to the design phase, including lab studies and field trials, enabled the grouping of hundreds of products based on formulation characteristics. The field trials also incorporated visual inspection, TOC, and microbial surface testing. These results were analyzed and leveraged to reduce the testing scheme during the validation and monitoring phases. Understanding the equipment design, spray coverage, flow dynamics, heating ramp rates, rinse, and drying conditions is critical to optimize field trials and improve the laboratory test model. The result is a validated cleaning process based on a scientific, risk-based approach for a wide range of products and the ability to quickly evaluate and place new product formulations within an appropriate cleaning family.
Acknowledgments
A special acknowledgment to Jennifer Gaffney, Mary Kay Global Director of Validation, for expertise and leadership throughout this project. The authors wish to extend their thanks to Haydeé del C. Rivera Alvelo for helping with the project.