Master Soil Selection for Cleaning Validation of Parts Washers

One of the goals of the cleaning validation design phase is to define critical process parameters (inputs) and acceptance criteria (outputs) of the cleaning process. This article explores the selection of a master soil as part of the cleaning validation design phase for automated parts washers. The selection and qualification of a master soil through laboratory testing and during factory acceptance testing (FAT) can be leveraged during onsite qualification to reduce the time and cost of cleaning validation processes.
It is standard practice in the industry for cleaning validation and equipment validation to embrace the process life-cycle three-stage model (design, qualify, and monitor).1, 2 The integration of cleaning process design testing with factory acceptance testing execution and cleaning validation of automated parts washers has been well documented.3, 4, 5 Standard performance tests that can be performed during factory acceptance testing include coverage and cleaning tests, which can be leveraged during commissioning and validation of the automated parts washer.5
A coverage test uses riboflavin (at approximately 0.2 g/L in water) as the test residue due to its ultraviolet (UV) fluorescence at 385–395 nm and high solubility in water, and UV light as an inspection method. The clean parts are then inspected by various methods, such as conductivity, UV spectroscopy, or total organic carbon (TOC). The main advantage of performing these tests during factory acceptance testing is that the automated parts washer or accessories can be modified at the factory, as opposed to the final location.
Working with or sending process residues to the equipment manufacturer is not always possible or practical. Challenges may include:
- Unavailability of process residue
- Testing restrictions from the manufacturer
- Cost of process residue
- Environmental restrictions
- Health and safety concerns for the operators
- Shipping regulations
- Confidentiality risks
To reduce the time and cost of multiple cleaning validations, a grouping or bracketing exercise can be performed to select the worst-case product, active ingredient, or process residue. Grouping products, such as drugs, and selecting a worst-case product, which is manufactured on the same equipment and cleaned by the same method, is well accepted by US FDA, Health Canada, Pharmaceutical Inspection Convention and Pharmaceutical Inspection Co-operation Scheme (PIC/S), ICH, WHO, and other regulatory agencies.6, 7, 8, 9, 10, 11
Master Soil Selection
A master soil is a surrogate residue and can be considered to be either a representative process residue or worst-case process residue, depending on the selection criteria. The worst-case soil can vary based on the equipment train or cleaning method used. A worst-case residue is generally selected based on a risk-based approach. Common factors considered include cleanability, solubility, toxicity, and availability. If the worst-case residue is sent to the equipment manufacturer, environmental hazards, operator health and safety, and shipping concerns also need to be considered.
Other factors to consider in master soil selection include the analytical method support, absence of animal-derived material, understanding of the rinse profile, defined soil characteristics, availability, cost, and a visible residue limit (VRL). For example, bovine serum can be easy to attain, low cost, and difficult to clean; however, it is an animal-derived material, is complex, and has an undefined residue. Mineral oil can be difficult to clean, is free of animal-derived material, is simple, and has a defined residue; however, discharging it into a municipal drain may not be acceptable. Refer to Table 1 for a list of possible master soils and factors to consider during master soil selection. The list of proposed master soils is based on literature related to pharmaceutical, biopharmaceutical, and medical device master soil selection and cleaning applications,12, 13, 14, 15, 16 as well as the authors’ industry experience. The selection of a master soil within a manufacturing facility may be a long process, but the efforts may be worthwhile due to the resource and cost savings achieved during qualification and validation.
Laboratory Evaluation
Figure 1 shows the application of a master soil to laboratory glassware. Laboratory testing has been effective at defining critical cleaning parameters for removing process residues,17, 18 and it is the best way to develop successful standard operating procedures (SOPs). The following is an example of steps in a laboratory test procedure:
- Weigh dry, clean 304 stainless steel coupons (7.5 × 15 cm) on an analytical balance (±0.1 mg) to obtain the precoating weight.
- Coat coupons coated with 3–5 mL of the sample. The amount of residue per surface area is controlled and recorded; it varies by the application form (dry powder, compressed powder, or slurry).
- Air-dry the samples at ambient temperature.
- Weigh the conditioned coupons on an analytical balance to determine precleaning weight.
- Clean each coupon by agitated immersion, spray wash, or cascading flow.
- Remove each coupon and visually observe it for cleanliness.
- Rinse each side of the coupon with tap water for 10 seconds at a flow rate of 2 L/min.
- Rinse each side of the coupons with deionized water and examine for a water break–free surface.
- Dry coupons and then weigh them on an analytical balance to determine the postcleaning weight.
Cleaning parameters for evaluation include the selection of the cleaning agent, time, temperature, and cleaning agent concentration. These specified cleaning parameters are confirmed with scale-up or field evaluations.
Master Soil | Availability | Ease of Cleaning |
Solubility in Water (at 25°C) |
Toxicity (Oral LD50 Rats) |
Complexity (Defined– Undefined) |
Environmental Risks |
Operator Safety Risks |
VRL |
---|---|---|---|---|---|---|---|---|
Albumin, bovine | 1 | 3 | 1 | 1 | 3 | 1 | 1 | 1 |
Aluminum phosphate | 1 | 1 | 2 | 1 | 1 | 2 | 1 | 2 |
Browne residue | 2 | 3 | 1 | 1 | 3 | 1 | 1 | 2 |
Egg, chicken | 2 | 3 | 2 | 1 | 3 | 1 | 1 | 1 |
Hemoglobin | 2 | 3 | 1 | 1 | 2 | 1 | 1 | 1 |
Ibuprofen | 2 | 1 | 2 | 1 | 1 | 1 | 1 | 2 |
Lactose | 1 | 2 | 1 | 1 | 2 | 1 | 1 | 1 |
Magnesium stearate | 1 | 2 | 3 | 1 | 1 | 1 | 1 | 2 |
Mineral oil (petroleum) | 1 | 2 | 3 | 2 | 1 | 2 | 1 | 1 |
Phosphate-buffered saline (PBS) |
1 | 1 | 1 | 1 | 2 | 1 | 1 | 3 |
Sucrose | 1 | 2 | 1 | 1 | 1 | 1 | 1 | 1 |
Sodium bicarbonate | 1 | 1 | 1 | 1 | 1 | 1 | 1 | 1 |
Cornstarch | 1 | 2 | 2 | 1 | 2 | 1 | 1 | 2 |
Tryptic soy broth (TSB) | 1 | 2 | 1 | 1 | 3 | 1 | 1 | 2 |
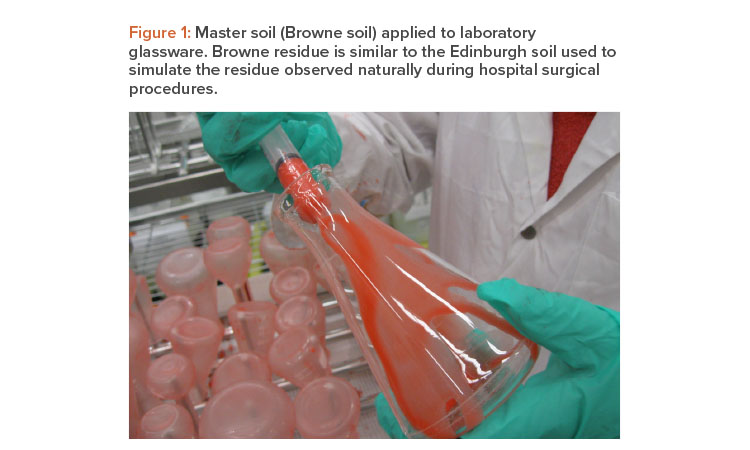
To evaluate different factors, such as safety, environmental impact, ease of cleaning, and the cost of raw materials, we elected to investigate the following soils for consideration as a master soil: bovine albumin, aluminum phosphate, Browne residue, egg (chicken), hemoglobin, ibuprofen lactose, magnesium stearate, mineral oil, phosphate-buffered saline (PBS), sucrose, sodium bicarbonate, starch, and tryptic soy broth (TSB). These residues represent different industry segments, such as pharmaceutical (oral solid dose and parenteral), biopharmaceutical, and medical device. These industry segments or others may be subdivided into smaller groups for master soil selection and qualification.
Table 2 summarizes our laboratory findings. A coupon was considered clean if it was visually clean and water break–free, and if its precoating weight and postcleaning weight were equal (<0.1 mg residue per 7.5 × 15–cm coupon). It is important that the master soil and process soils can be cleaned to predetermined acceptance criteria, and the decision is not based on a percentage of residue removed.
Type of Cleaning Agent | |||||
---|---|---|---|---|---|
Master Soil | Water Only | Acid Detergent | Neutral Detergent |
Alkaline Detergent | VRL, μg/cm2 |
Albumin, bovine | - | - | - | Yes | 2 |
Aluminum phosphate | - | Yes | - | - | 10 |
Browne residue | - | - | - | Yes | 10 |
Egg, chicken | - | - | - | Yes | 2 |
Hemoglobin | - | - | - | Yes | 2 |
Ibuprofen | - | - | Yes | Yes | 10 |
Lactose | - | - | Yes | Yes | 2 |
Magnesium stearate | - | - | Yes | Yes | 10 |
Mineral (petroleum) oil | - | - | Yes | Yes | 1 |
PBS | Yes | Yes | Yes | Yes | 100 |
Sucrose | Yes | Yes | Yes | Yes | 1 |
Sodium bicarbonate | - | Yes | - | Yes | 1 |
Starch, maize | - | - | Yes | Yes | 10 |
TSB | - | - | - | Yes | 10 |
*The yellow areas represent a high-temperature cleaning condition. “Yes” indicates that the residue was successfully cleaned with the cleaning agent. A dash represents a cleaning failure. VRL is the quantity of residue on stainless steel that can be seen by the inspector; it is determined through visual inspection and control of critical parameters.
As a part of the cleaning evaluation, we also explored VRLs for the proposed master soils. Visual inspection is usually the first step in determining whether the equipment is clean before scheduling analytical testing. In 1993, Fourman and Mullen specified a visual limit for small molecule active ingredients of 1–4 μg/cm2,19 and this article is referenced in the US FDA “Guide to Inspections Validation of Cleaning Processes”11 as well as Par-enteral Drug Association Technical Report 29.20 Forsyth and colleagues published at least one article that includes testing and definitions of critical variables and presents case studies.21 The case studies include spiking a 1 cm2 surface with residue at various concentrations and on different substrates. Once the residue is dried, it can then be inspected visually at different distances, angles, and light intensities, with the use of mirrors and by different analysts.21, 22, 23 By defining the operators’ qualifications, visual inspection tools and conditions, procedures, training, and retraining activities, a company can quantify and validate the visual inspection procedure.23
The VRL study procedure was employed as follows:
- Coupons made of 304 stainless steel with 2 B finish were precleaned.
- Test master soil samples were serially diluted at 1 μL and 20 μL of low-TOC water and applied over a 1 cm2 area of each coupon.
- Samples were air-dried for 16 hours.
- Coupons were inspected in duplicate (by two analysts) at one of the following distances (0.45 meter, 1.0 meter, or 1.5 meter), lighting conditions (250 lux, 500 lux, or 1,000 lux), viewing angle (30°, 45°, or 90°), and with and without a viewing mirror. A Cooke Corporation Cal-Light 400-lux meter, digital protractor, and Hamilton 10-μL syringes were used in the study.
- The VRL results were reported (Table 2).
Equipment Evaluation
The first step in an optimal rack design evaluation is to collect information on the parts that need to be cleaned, such as materials of construction, dimensions and shapes, type of process soil, and condition of the soil on the surface.4 This information is critical in developing a grouping strategy, de-signing the cleaning cycle, and defining the loading configuration of the parts. Once this information is gathered, the loading configuration can be established, and laboratory cleaning evaluations can be performed to support cycle development. Figures 2 and 3 show how parts may be loaded in a washer rack.
Grouping or bracketing of items is important in testing worst-case load configurations during this design stage. A single process soil can be cleaned (as would be the case with filling equipment), or several soils can be washed in a single cycle. The cleaning cycle needs to be effective at removing residues. To demonstrate cleanliness, the residue is evaluated when selecting the analytical method and setting acceptance criteria. Once the loading configuration is defined, loading rack options should be investigated to determine what could be suitable based on the parts list and grouping strategy.
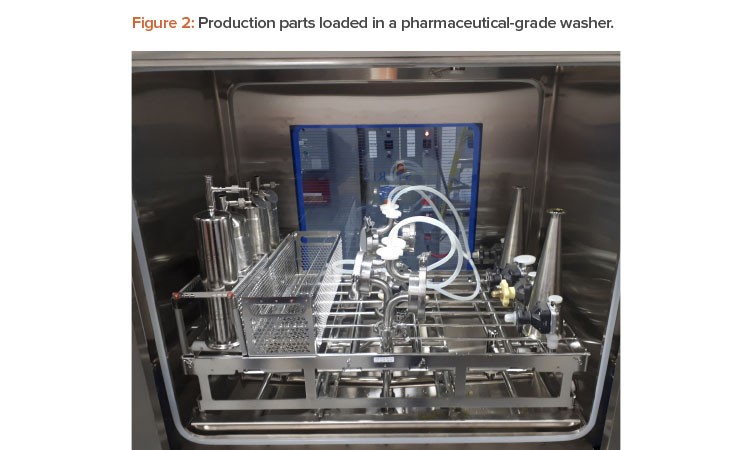
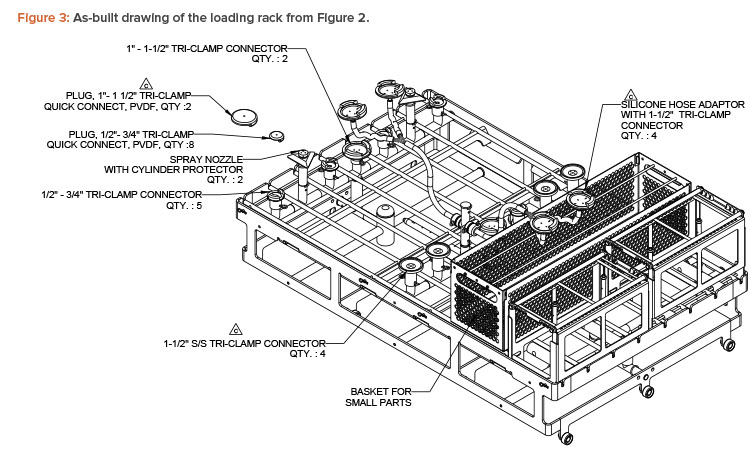
After loading patterns are identified and racks are designed and assembled, it is important to verify that the rack design allows proper coverage on process parts. To do so, equipment manufacturers can conduct coverage tests using riboflavin diluted at 0.2 g/L, or another solution. Components are soiled by spraying or gently misting the solution onto the interior and exterior surfaces.24 (See Figure 4 for an example of the soiling process.) The main objective of coverage testing is to assess coverage efficacy of standard or customized racks and associated process parts. This field trial ensures that water reaches all surfaces, items remain secure in the rack, and any soluble cleaning agent used can be successfully rinsed. This performance test is normally done as part of FAT and should ideally be conducted with the parts that are used onsite, or representative parts.
In washing applications, coverage is the most important critical cleaning parameter because incomplete coverage means that the cleaning solution does not reach internal and external surfaces of the components to be cleaned. Some parts, like tubing or hoses, are typically harder to clean because of their surface geometry and higher flow velocity requirements. Coverage efficacy is determined by visual evaluation of remaining soil on glassware and parts. No detergent is added to the cycle, and only water is used. Dry time can vary between seconds and hours, depending on preference. It is important to mention that coverage testing is not a cleaning challenge; therefore, the solution should be water soluble and easily removable from the surface.
Before spraying the actual parts with riboflavin or other soiling solution, apply a small quantity on a stainless steel surface and use a UV light to verify that the solution fluoresces. After verification, spray the parts and rack and load them into the washer for a short rinse phase. Parts can then be unloaded from the washer chamber and immediately inspected with a UV light for traces of riboflavin (Figure 5). It is important to inspect areas that are harder to reach. Modifications may be made to the rack following a coverage test to improve parts orientation and, therefore, coverage.
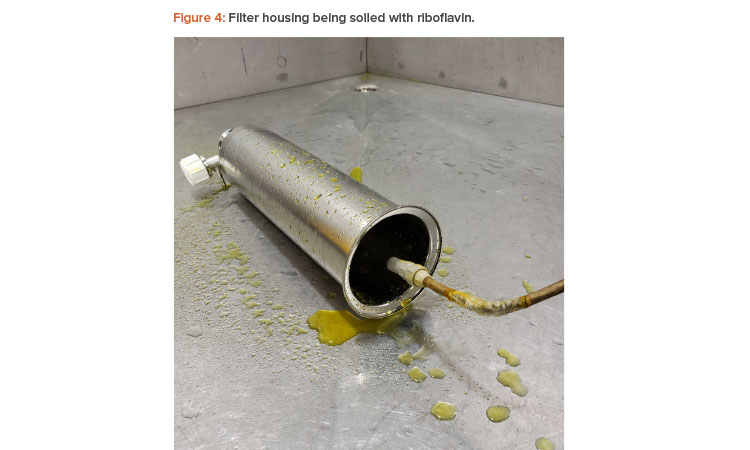
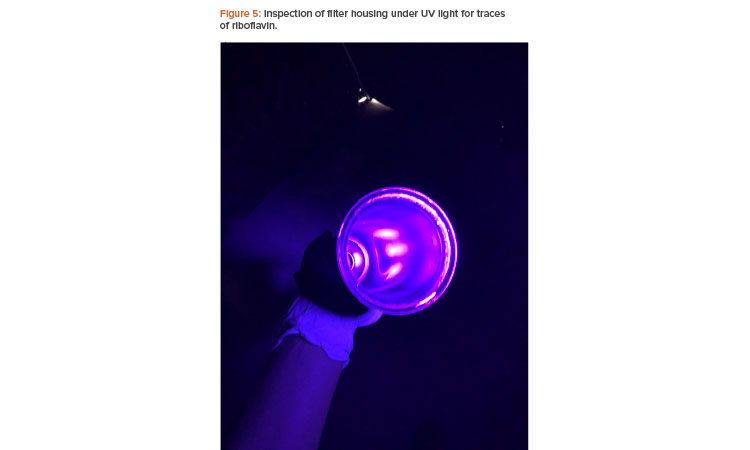
Application of Master Soil
The master soil can be applied to perform a cleaning test using a similar technique as the riboflavin test described previously. The master soil could also be used in combination with the riboflavin; however, omitting the initial coverage test using riboflavin only is not recommended. As noted earlier, the coverage test is important to confirm that all areas are wetted during the rinse step. This test helps identify potential areas to sample as well as significant engineering concerns.
The cleaning test is performed using a standard or normal parts washer cycle. This cycle may include a prerinse, a series of wash steps, post-wash-step rinses, a final rinse, and a heated drying step. During the cleaning test, the washer cycle should run uninterrupted and without alarms. Based on the authors’ experience, adding riboflavin to the proposed master soil does not adversely affect the cleaning parameters of these residues. Adjusting the master soil concentration or conditioning process can significantly change the cleaning parameters required to remove the residue.
Upon completion of the parts washer cycle, the cleaned parts can be visually inspected to confirm the removal of the master soil to acceptable levels (refer to the earlier discussion on VRLs). Additional swabbing of the parts or rinse-water testing may be warranted based on the sensitivities of the residue and detection method. The analytical testing methods available to equipment testing facilities during FAT may be limited; therefore, it is important to have confidence in the quality of visual inspection or rinse-water analysis.
Case Study
A process development and cGMP manufacturing facility of recombinant proteins for a large multinational company was interested in identifying a master soil that would be more difficult to clean than their worst-case process residue and could be used for cleaning cycle development and validation. The process soils included final inclusion bodies, acidified/clarified protein (diethylaminoethyl [DEAE] load), bulk drug substance A (BDS-A), and bulk drug substance B (BDS-B). The master soils for screening included xanthan gum, starch, TSB, and soy protein isolate. The selection of the master soils for screening included the following criteria: availability, solubility in water, low toxicity, low complexity, no animal-derived material, no dyes, no perfumes, low operator safety, ease of detection, ease of soil conditioning, and a worst-case cleaning procedure compared to the process soils.
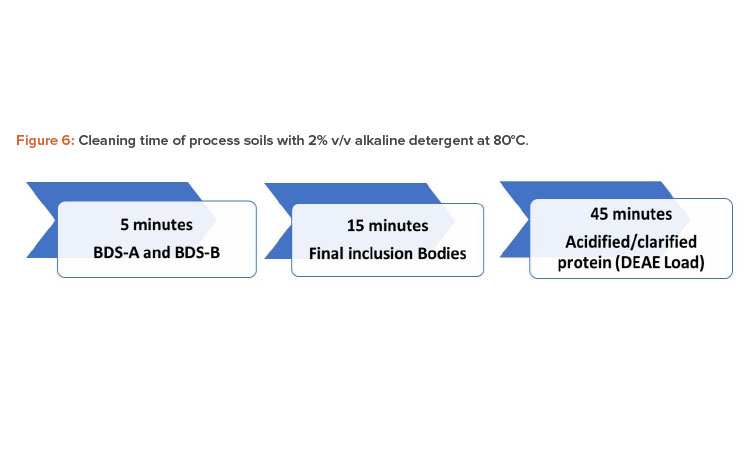
The cleaning of the process soils and master soils at various percent weight-per-volume (% w/v) concentrations were performed following the procedure described previously. The dirty hold time for the process and master soils was more than 16 hours at ambient temperature. The process soils were cleaned within 45 minutes using a 2% volume/volume (v/v) dilution of an alkaline detergent at 80°C, as detailed in Figure 6. The starch, TSB, and soy protein isolate at 3% and 6% w/v were cleaned within 5 minutes. At 1% w/v, xanthan gum was cleaned within 15 minutes, and at 3% w/v, it was cleaned within 45–60 minutes. Xanthan gum at 3% w/v was selected as the master soil.
Conclusion
Regulatory guidance documents and published industry best practices agree that grouping or bracketing of residues and the use of placebo or master soils are acceptable with justification. In the review of FATs conducted over the past few years, the authors found that almost all end users would elect to include a coverage test during an automated parts washer FAT, but almost no FATs included a cleaning challenge. The work performed during the FAT is generally repeated during the site acceptance testing and then again during the installation qualification and operational qualification of the washer. This practice leads to delay and risk when advancing to the performance qualification and cleaning validation of the automated parts washer.
The risk can be reduced by performing laboratory studies. For example, coupon studies (as described earlier) can help define critical cleaning parameters (inputs) and acceptance criteria (outputs) before testing with the washer is performed. This is a sound scientific approach, which would be improved if a placebo or master soil were included as part of the laboratory study and as part of FAT of the automated parts washer. This approach also supports the inclusion of analytical process tools, such as inline conductivity and TOC testing of rinse water.25, 26